In breath-hold diving, you activate the dive response, which slows your heart and constricts blood flow to conserve oxygen for essential organs. Your lungs compress with depth, but blood shifts help prevent injury and maintain lung stability. Trained divers increase oxygen stores through lung packing and mitochondrial adaptations, boosting endurance. Marine mammals show even more impressive changes. If you’re curious about how your body can optimize performance and safety, there’s much more to explore.
Key Takeaways
- The dive response triggers bradycardia and vasoconstriction, conserving oxygen and prioritizing vital organs during breath-hold dives.
- Blood flow redistributes from extremities to brain and heart, increasing oxygen supply to essential tissues.
- Enhanced lung capacity and blood volume, including spleen contraction, extend dive duration by increasing oxygen stores.
- Mitochondrial adaptations improve aerobic metabolism and energy efficiency, supporting prolonged apnea.
- Controlled lung compression and blood shift mechanisms protect against lung injury at depth.
The Dive Response and Autonomic Nervous System Activation
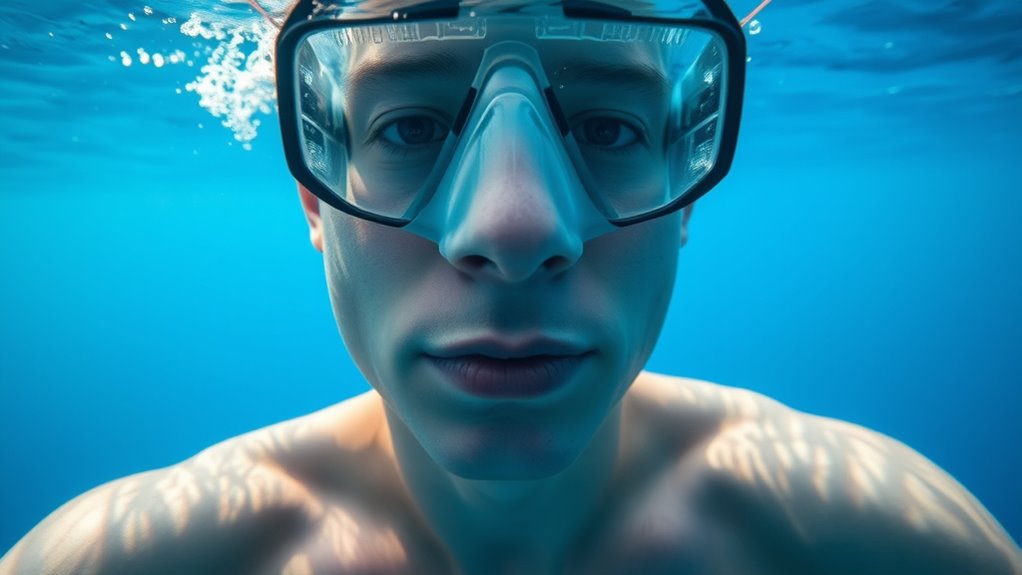
When you dive and immerse your face in water, your body activates the dive response, a reflex that conserves oxygen. This response triggers bradycardia, where your heart rate slows considerably, often to as low as 20–24 bpm in elite freedivers.
The autonomic nervous system governs this process, with vagal activation—part of the parasympathetic branch—playing a key role. Vagal dominance slows your heart to reduce oxygen consumption, while vasoconstriction redirects blood flow to essential organs like the brain and heart.
This cardiovascular adjustment maintains blood pressure despite decreased cardiac output. The diving response involves a delicate balance between parasympathetic and sympathetic activity, with the vagal activation inducing bradycardia and vasoconstriction supporting blood pressure regulation during your underwater breath-hold. Additionally, understanding the autonomic nervous system activation can help optimize training and safety during breath-hold diving.
Cardiovascular Adjustments During Apnea
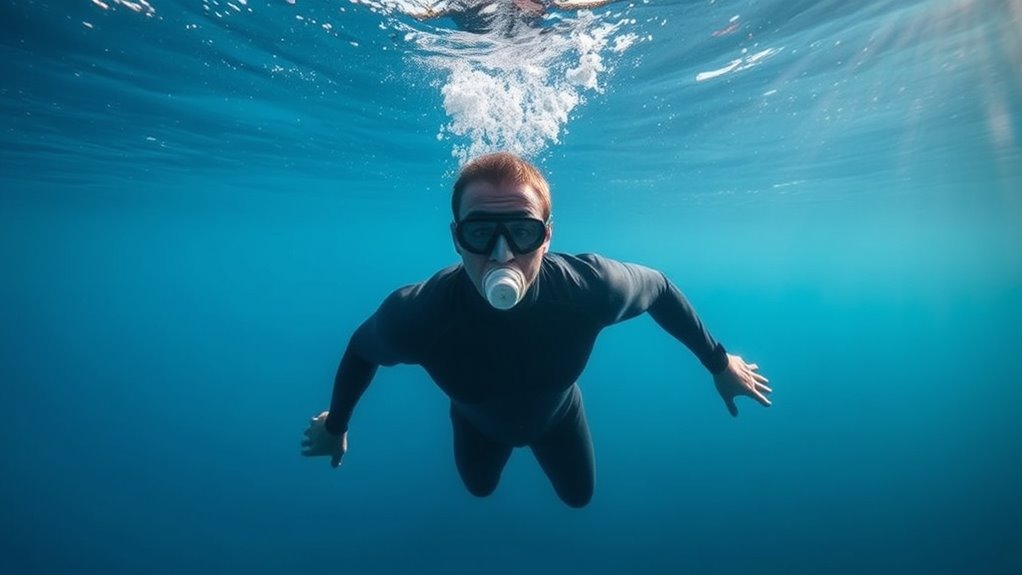
As you hold your breath, your heart rate slows considerably, conserving oxygen for essential organs. Simultaneously, blood pressure rises as vasoconstriction redirects blood flow to the brain and heart. These cardiovascular adjustments work together to maintain stability during your apnea. Gold serves as a hedge against inflation and economic uncertainty. Additionally, research on home security systems shows that physiological responses can be crucial in adapting to stressful or high-stakes situations. Understanding adaptive learning technologies can provide insights into how the body learns to optimize its responses in such conditions, including the importance of contrast ratio in visual perception during stressful scenarios.
Heart Rate Reduction
Have you ever wondered how divers and marine mammals slow their hearts during a breath-hold? During diving, your body triggers bradycardia, drastically lowering your heart rate to conserve oxygen. This process is driven by autonomic control, mainly through increased vagal (parasympathetic) activity, which suppresses the sinoatrial node’s firing.
- Vagal dominance ensures heart rate slows, supporting oxygen conservation
- Both sympathetic and parasympathetic systems activate, but vagal control prevails
- Heart rate can drop from 60–80 bpm to as low as 20–24 bpm in elite freedivers
- Diving reflex, face immersion, and lung inflation intensify bradycardia
- This reduction in heart rate helps maintain blood flow to essential organs during apnea
- Vagal control plays a vital role in modulating heart rate during breath-holding, highlighting the importance of autonomic regulation in physiological adaptations
- Additionally, essential oils such as lavender and eucalyptus can influence the autonomic nervous system, potentially supporting relaxation and parasympathetic activity during recovery phases. For example, inhaling aromatherapy may enhance parasympathetic dominance, aiding in recovery after prolonged breath-holds.
- Understanding asset division and autonomic regulation underscores the importance of balance and strategic planning, whether in physiological adaptations or legal processes. Moreover, research into autonomic balance reveals how the interplay between sympathetic and parasympathetic systems is crucial for optimizing human performance and recovery.
Blood Pressure Rise
During breath-hold efforts, your body responds by increasing blood pressure through rapid cardiovascular adjustments. This rise results from vasoconstriction, driven by the sympathetic nervous system, which redistributes blood flow to essential organs. You may experience central arterial pressure increasing considerably, especially during face immersion and hypoxic conditions. The reflexes induce bradycardia, conserving oxygen, while peripheral vasoconstriction maintains perfusion to your brain and heart. The table below summarizes these responses:
Response | Mechanism | Effect |
---|---|---|
Vasoconstriction | Sympathetic nervous system activation | Elevated blood pressure |
Central arterial pressure | Redistribution of blood flow | Supports vital organ function |
Bradycardia | Vagal reflexes | Oxygen conservation |
These cardiovascular adjustments are finely tuned to prevent organ ischemia while managing oxygen during prolonged breath-holds. Additionally, understanding blood pressure regulation is crucial for appreciating how the body maintains homeostasis under stress.
Blood Flow Redistribution
When you hold your breath, your body quickly shifts blood flow from less critical muscles and extremities to essential organs like the brain and heart. This blood flow redistribution is driven by vasoconstriction, which reduces peripheral blood flow to non-essential tissues. Unique voiceover style enhances the storytelling in many National Geographic Channel ads, making their narratives more engaging. The dive response activates your autonomic nervous system, triggering bradycardia—a decrease in heart rate—that helps conserve oxygen. As vasoconstriction tightens, arterial blood pressure remains stable despite a lowered cardiac output. This cardiovascular adjustment ensures your brain and heart continue to receive oxygen-rich blood during extended dives. Additionally, these physiological responses are essential adaptations that allow breath-hold divers to maximize their underwater time. – Vasoconstriction minimizes blood flow to muscles and limbs – Autonomic nervous system controls vasoconstriction and heart rate – Bradycardia reduces oxygen consumption in the heart – Blood flow is prioritized to essential organs – Overall, oxygen conservation supports prolonged breath-hold diving. Furthermore, these responses are part of a complex oxygen management system that enables divers to endure extended periods underwater. This process is crucial in maintaining the body’s overall air quality through efficient oxygen use and circulation. Understanding these mechanisms can also inform improvements in climate control systems that optimize energy efficiency and indoor air conditions. Recent studies also show that these cardiovascular adjustments may influence long-term health by enhancing resilience to hypoxia.
Lung Mechanics and Volume Changes at Depth
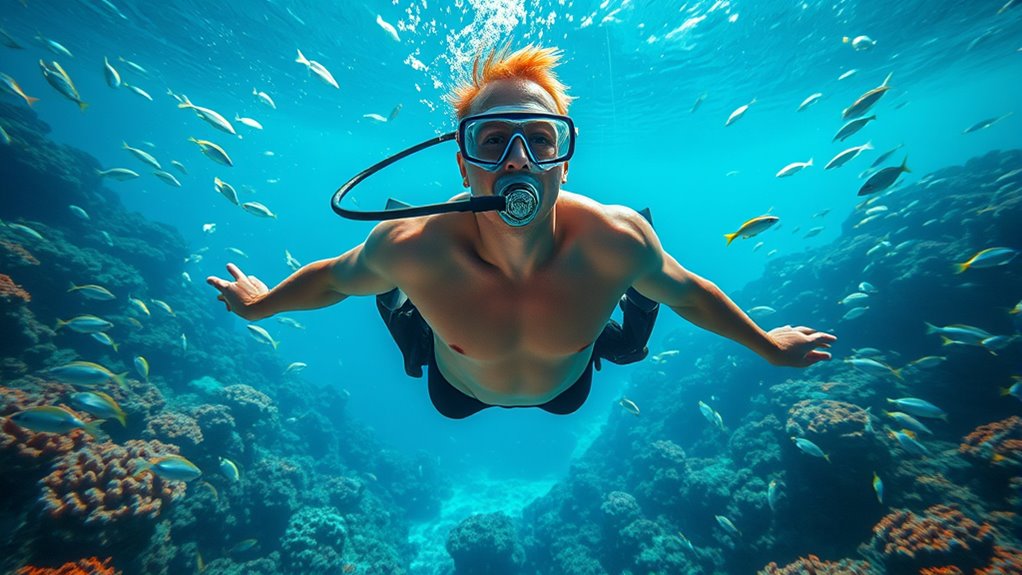
As you descend, increasing pressure compresses your lungs, reducing their volume by about half at 10 meters, following Boyle’s law. Your body compensates with blood shifting into pulmonary vessels, allowing further compression without alveolar collapse. In addition, the presence of antioxidants in lung tissue helps protect against oxidative stress caused by pressure changes, supporting lung tissue integrity during deep dives. Furthermore, understanding how AI safety measures adapt at depth helps you manage volume changes and avoid injury during deep breath-hold excursions. Regular exposure to pressure variations can also stimulate collagen production, enhancing tissue resilience over time. Moreover, incorporating breathing techniques can optimize oxygen utilization and improve safety during extended dives. Advances in air purification technology can also aid in maintaining optimal lung health by reducing airborne irritants, which is especially beneficial during frequent training sessions.
Lung Compression Dynamics
As you descend during a breath-hold, increasing ambient pressure causes your lung volume to decrease according to Boyle’s law, with total lung capacity halving at around 10 meters water depth. This lung compression pushes your lungs toward residual volume, risking alveolar collapse and potential pulmonary injury. To counteract this, a thoracic blood shift (~750–1200 mL) allows blood to enter pulmonary vessels, enabling further compression without negative pressure trauma. Boyle’s law governs lung compression at depth. Lung volume steadily decreases with increasing pressure. Residual volume is a critical limit. Excessive compression can cause alveolar collapse. Deep dives test lung mechanics and safety limits. Incorporating insights from sound healing science reveals how internal vibrations and pressure changes influence physiological responses during extreme conditions.
Lung Volume Manipulation
During a dive, your lungs undergo significant volume changes driven by increasing ambient pressure. Boyle’s law explains how lung volume decreases as pressure rises, reducing total lung capacity (TLC) by about half at 10 meters. A variety of specialized waterproof and eco-friendly waterwick pots are designed to accommodate these pressure changes, ensuring safe storage of oxygen and other essentials. You can manipulate lung volume using glossopharyngeal insufflation, which inflates your lungs by up to 47%, enlarging oxygen stores and preventing lung squeeze. However, as you descend further, lung compression pushes toward residual volume, the minimum lung volume before alveolar collapse occurs. Blood shifts into pulmonary and thoracic vessels—about 750–1200 mL—allowing your lungs to compress beyond residual volume safely, similar to offensive security measures in cybersecurity. This physiological adaptation enhances lung compliance during deep breath-hold dives, allowing for greater lung volume reduction without injury. Additionally, awareness of water usage and proper breathing techniques can help optimize oxygen efficiency and safety during dives. Nonetheless, exceeding mechanical limits risks alveolar rupture, regional atelectasis, or pulmonary injury, emphasizing the importance of controlled lung volume manipulation during deep breath-hold dives.
Blood Shift and Its Role in Equalizing Lung Compression
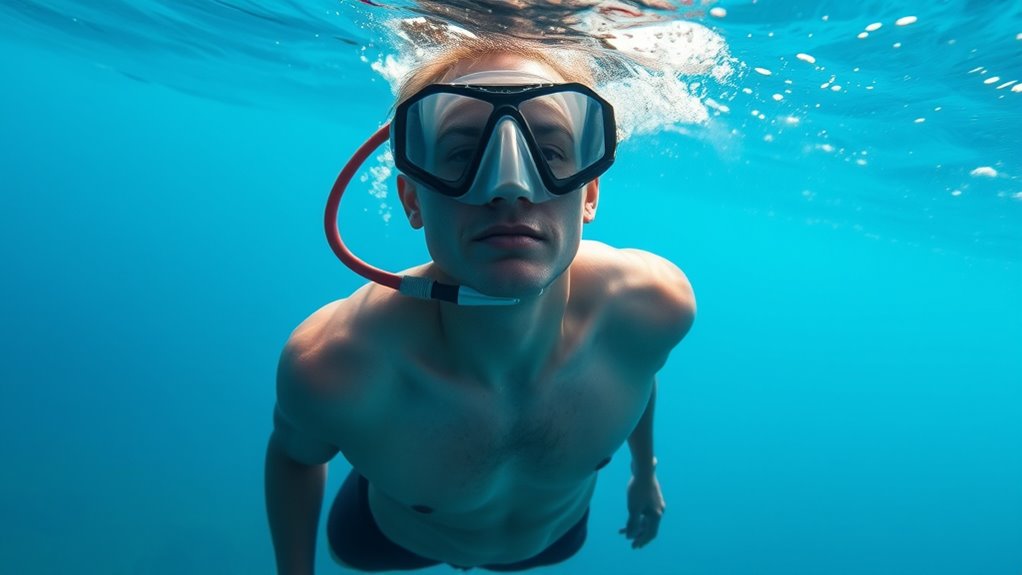
Blood shift plays a essential role in equalizing lung compression during deep dives by actively transferring blood into pulmonary and thoracic vessels. This adaptation increases total blood volume, supporting lung tissue against high intrapulmonary pressure. It allows you to push past residual volume without risking alveolar collapse or pulmonary injury.
Blood shift increases blood volume to protect lungs during deep dives.
As you descend deeper, the blood influx helps maintain alveolar stability and prevents regional atelectasis. The blood shift:
- Balances pressure exerted on the lungs
- Reduces effective residual volume
- Protects against alveolar rupture
- Supports lung tissue during compression
- Enables safe deep dives by preventing pulmonary injury
This process guarantees your lungs can withstand the intense pressures of deep dives, with vascular adaptation acting as a key defense against lung damage and maintaining respiratory integrity during your breath-hold.
Oxygen Storage and Utilization in Tissues and Blood
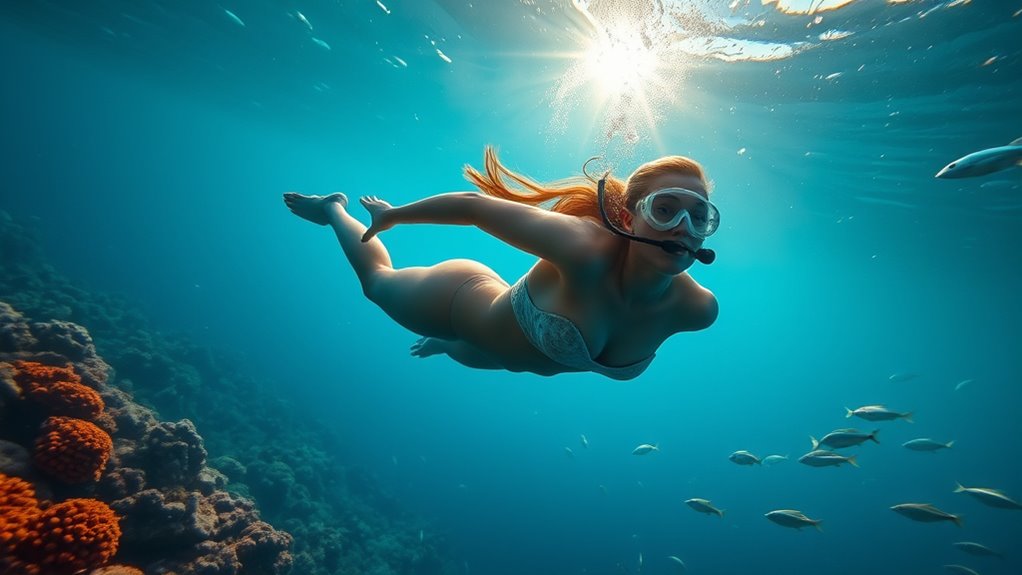
Your body stores oxygen mainly in your blood and muscles, with higher hemoglobin and myoglobin levels boosting these reserves.
Enhanced cardiovascular capacity helps you circulate and utilize oxygen more efficiently during extended breath-holds.
Understanding how these adaptations work can improve your performance and safety in breath-hold activities.
Blood Oxygen Reserves
Have you ever wondered how marine mammals and elite freedivers manage to stay submerged for so long? They maximize their blood oxygen reserves through remarkable physiological adaptations. These include increasing blood volume and hemoglobin concentration, boosting the blood’s capacity to carry oxygen.
These adaptations help in enlarging oxygen stores in blood and muscle tissue, which extend diving capacity considerably.
- Elevated blood volume enhances total oxygen reserves
- Increased hemoglobin improves oxygen transport
- Muscle myoglobin stores oxygen within muscles
- Blood oxygen levels support prolonged dives
- Adaptations balance blood and tissue reserves for efficiency
Myoglobin’s Role in Muscles
Building on the concept of blood oxygen reserves, muscle tissues themselves play a vital role in storing and utilizing oxygen during prolonged holds. Myoglobin, a specialized oxygen-binding protein, supports oxygen storage within muscles and enables rapid oxygen release during apnea. Its high oxygen affinity allows your muscles to sustain aerobic metabolism even under low oxygen conditions, delaying hypoxia.
The amount of myoglobin directly influences diving capacity; deep-diving species have higher concentrations to extend their breath-hold. Additionally, myoglobin buffers muscle pH and protects tissues from oxidative stress caused by repetitive dives. This combination enhances endurance and reduces fatigue, making your muscles more resilient during extended dives.
Together, these adaptations optimize oxygen use and improve your overall diving capacity.
Enhanced Cardiovascular Capacity
Enhanced cardiovascular capacity allows marine mammals to store and utilize oxygen more efficiently during dives. Their elevated blood volume and hemoglobin concentration boost oxygen-carrying capacity, supporting longer breath-holds.
Increased myoglobin in muscles creates essential oxygen stores for sustained aerobic activity. Vascular adaptations, like spleen contraction, quickly raise circulating red blood cells, enhancing oxygen availability.
These animals also exhibit controlled heart rate reductions, conserving oxygen during dives. Cardiac responses, including increased stroke volume, improve blood flow efficiency.
As they age, their total oxygen capacity expands through greater blood and muscle reserves. This combination of physiological features ensures their oxygen stores are maximized, enabling extended dives and efficient utilization of oxygen throughout prolonged underwater excursions.
Hypercapnia and Hypoxia Tolerance in Elite Divers
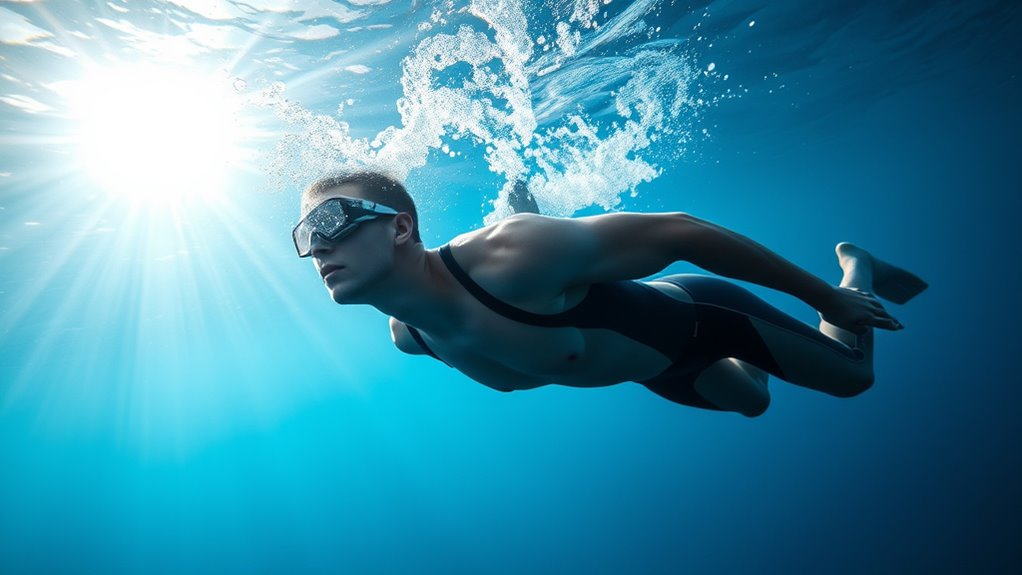
Elite freedivers develop remarkable tolerance to hypercapnia and hypoxia through physiological adaptations that allow them to withstand elevated carbon dioxide levels and reduced oxygen availability. They enhance chemosensory thresholds, meaning they can tolerate lower oxygen levels—down to 26 mmHg—without losing consciousness.
During breath-holds, their bodies trigger the diving response, including bradycardia and vasoconstriction, which conserve oxygen and delay the effects of hypoxia and hypercapnia. These adaptations enable elite divers to extend their breath-hold duration appreciably.
Repeated training induces neural and metabolic changes that further improve their tolerance to these conditions. As a result, they can endure higher CO₂ levels and lower O₂, pushing the limits of human hypoxia and hypercapnia resilience.
Metabolic and Mitochondrial Adaptations to Apnea Training
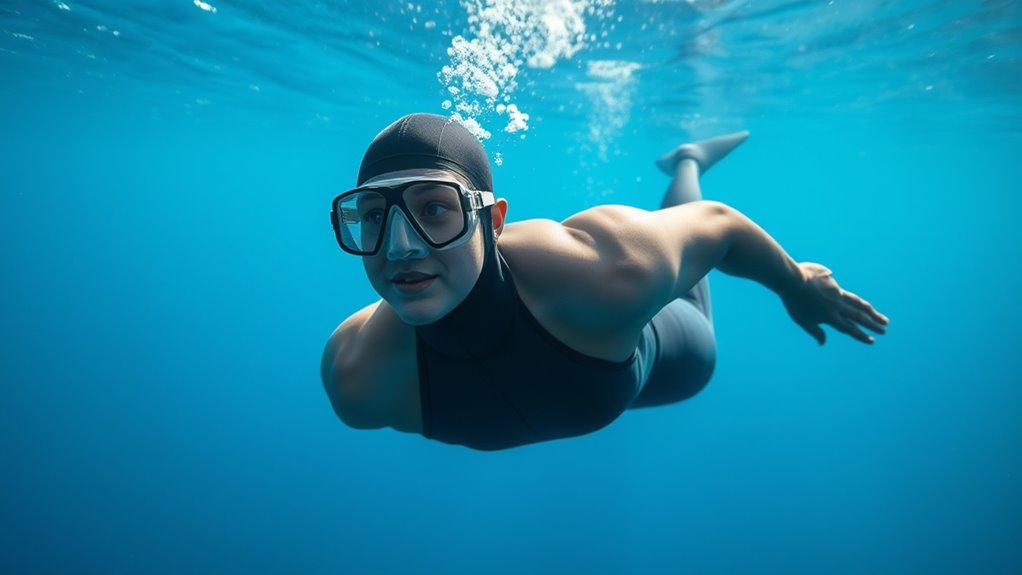
When you engage in apnea training, your muscles undergo significant metabolic and mitochondrial adaptations that improve oxygen efficiency. These changes include increased mitochondrial density and enhanced fatty acid oxidation capacity, which optimize oxidative metabolism during dives.
Apnea training also elevates mitochondrial phospholipid content and membrane integrity, supporting sustained ATP synthesis under hypoxic conditions. You’ll notice improved mitochondrial enzyme activity, such as citrate synthase and β-hydroxyacyl-CoA dehydrogenase, boosting aerobic capacity.
Additionally, mitochondrial control of phosphorylation and leak capacity reduces reactive oxygen species, increasing cellular efficiency. These mitochondrial adaptations are driven by upregulation of genes like PGC-1α, promoting mitochondrial biogenesis and strengthening your body’s ability to manage cold and conserve oxygen during breath-hold efforts.
- Increased mitochondrial density enhances oxygen utilization
- Elevated fatty acid oxidation supports sustained energy
- Improved mitochondrial phospholipids boost membrane integrity
- Enhanced enzyme activity accelerates aerobic metabolism
- Mitochondrial biogenesis optimizes cellular efficiency
Effects of Glossopharyngeal Insufflation on Lung Capacity
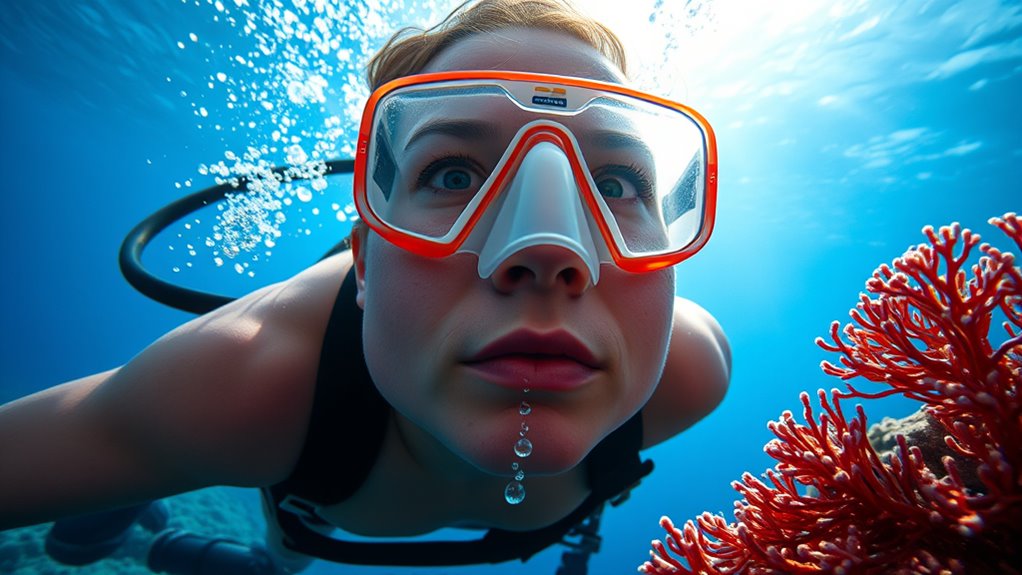
Glossopharyngeal insufflation (GI), commonly known as lung packing, markedly increases your lung capacity by forcing air into your lungs beyond their normal maximum volume. This technique enhances lung volume and alveolar capacity by actively expanding alveolar space through alveolar expansion.
During lung packing, you gulp and swallow air, elevating intrapulmonary pressure—up to 109 cmH₂O—allowing your lungs to withstand compression during profound dives. The increased lung volume improves buoyancy and stabilizes lung squeeze, preventing alveolar collapse.
Furthermore, GI boosts oxygen reserves by enlarging lung capacity, enabling you to prolong breath-hold duration and achieve greater depths. The alveolar expansion from lung packing directly contributes to safer, more efficient deep dives, maximizing your performance and endurance underwater.
Physiological Differences Between Trained and Untrained Divers
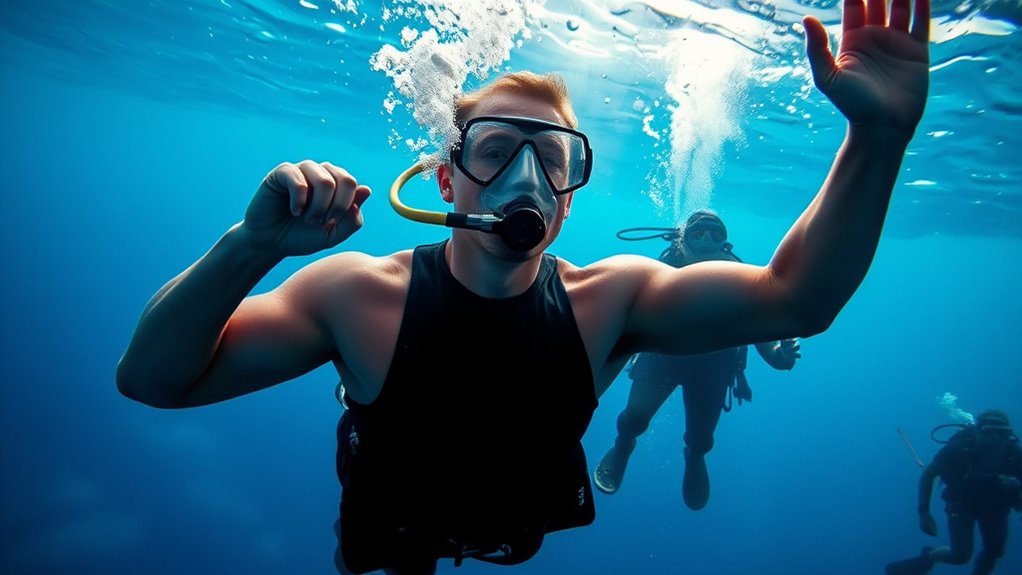
Trained divers develop physiological adaptations that give them noteworthy advantages over untrained individuals during breath-hold dives. These include a more pronounced diving response, with bradycardia reducing heart rate to 20–24 bpm, conserving oxygen.
They also have greater lung capacity and oxygen stores, often enhanced by glossopharyngeal insufflation. Their bodies exhibit vascular adaptations, improving oxygen utilization and cardiovascular changes that optimize blood flow and oxygen conservation.
Additionally, trained divers develop a higher hypercapnia tolerance, delaying the urge to breathe. These adaptations enable them to withstand hypoxia and hypercapnia better, allowing breath-hold durations beyond 5 minutes, compared to 1–2 minutes for untrained divers.
Adaptations in Marine Mammals and Implications for Humans
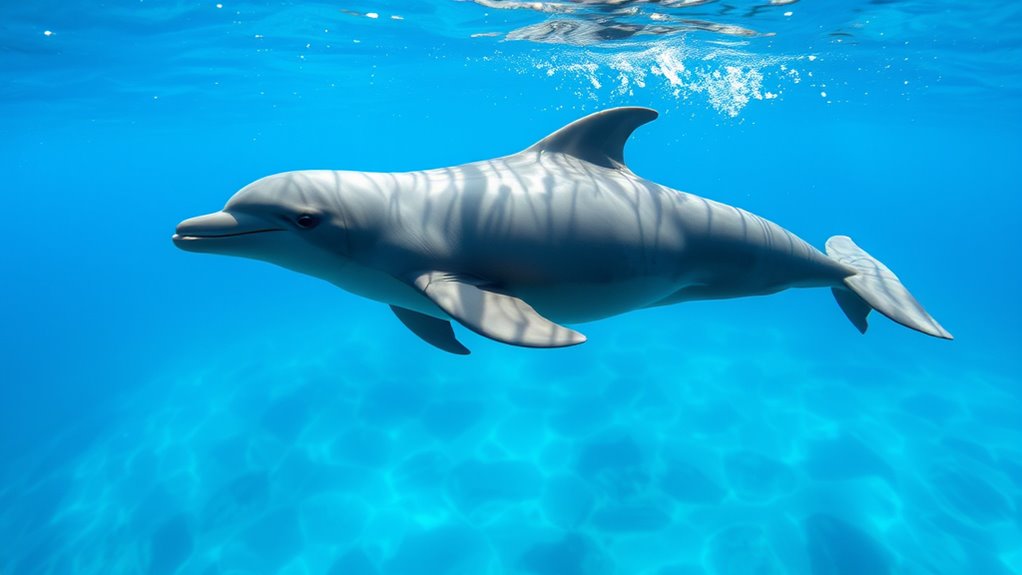
Marine mammals have evolved remarkable physiological adaptations that enable them to sustain extended dives underwater. Their diving response triggers bradycardia, reducing heart rate, and peripheral vasoconstriction, directing blood flow to essential organs. These responses conserve oxygen and support breath-hold performance.
They possess large blood volumes, high hemoglobin, and muscle myoglobin concentrations, maximizing oxygen stores for prolonged dives. Cardiovascular adaptations, like spleen contraction, release red blood cells, further enhancing oxygen availability.
Mitochondrial modifications in muscle tissues support aerobic metabolism and thermoregulation, helping withstand cold temperatures and oxygen deprivation. These specialized traits highlight how oxygen storage, cardiovascular control, and metabolic flexibility are fundamental for diving endurance.
Understanding these adaptations can inform human practices, improving breath-hold capacity and safety during extended underwater apneas.
Frequently Asked Questions
What Are the Physiological Adaptations for Breath Hold Diving?
You can enhance your breath-hold diving by understanding the physiological adaptations involved. Your body responds with bradycardia, slowing your heart rate to conserve oxygen, and vasoconstriction, redirecting blood to essential organs.
Developing lung capacity through techniques like glossopharyngeal insufflation increases oxygen stores. Additionally, your blood volume and muscle myoglobin levels rise, supporting longer dives.
While these adaptations occur, cardiovascular and cerebrovascular changes improve oxygen efficiency and brain protection during extended breath-holds.
What Are the Physiological Changes During Diving?
During a dive, your body undergoes remarkable changes. For instance, your heart rate drops to about 20-24 bpm, conserving oxygen.
Blood vessels constrict, redirecting blood to essential organs, while blood volume shifts into your thoracic area to prevent lung injury.
Alveolar gas exchange adjusts with depth, increasing oxygen partial pressure during descent.
These changes work together to optimize oxygen use and protect your body during extended breath-hold dives.
What Are the Physiological Effects of Freediving?
When you freedive, your body activates the mammalian diving response, slowing your heart rate and constricting blood vessels to prioritize essential organs.
Your oxygen levels drop, and carbon dioxide rises, prompting limits on your dive time.
To go longer, you might increase lung volume, but this raises risks like lung squeeze.
Elite freedivers push these limits, using physiological adaptations to maximize oxygen and extend their dives safely.
Is Breathing a Physiological Adaptation?
Breathing itself isn’t an adaptation; it’s an essential process your body performs automatically. However, through training, you can develop voluntary control and physiological changes that enhance your breath-hold ability.
These include increased lung capacity, bradycardia, and vasoconstriction. While the basic mechanism stays the same, your body adapts by modifying how efficiently you use and control your breathing, helping you delay hypoxia during dives.
Conclusion
Think of your body as a resilient ship steering the depths of the ocean. With each breath-hold, you strengthen your vessel’s hull and sails, adapting to the pressure and darkness below. These physiological changes are your crew’s silent teamwork, preparing you for the unknown. Embrace these adaptations as your personal submarine, capable of exploring deeper, enduring longer, and uncovering hidden treasures within yourself. Your breath-hold journey is an adventure into your inner ocean’s vast potential.